Scanning Transmission X-Ray Microscopy (STXM): Unveiling the Nanoscale World
What is Scanning Transmission X-ray Microscopy (STXM)?
Scanning Transmission X-ray Microscopy (STXM) is a powerful imaging technique that combines the principles of X-ray spectroscopy and microscopy to study the chemical composition, structure, and electronic properties of materials at the nanoscale. STXM uses a focused X-ray beam to scan the sample, providing high-resolution images with chemical sensitivity and elemental specificity.
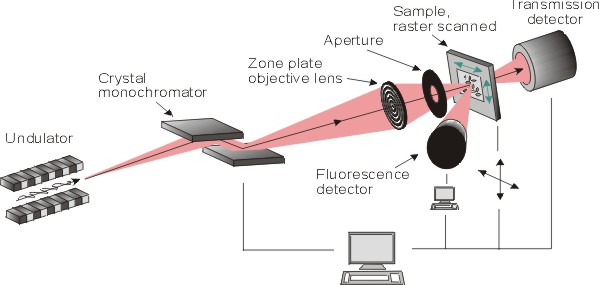
Key Components of STXM
A typical STXM setup consists of the following key components:
- X-ray Source: STXM typically uses synchrotron radiation as the X-ray source, which provides a high-intensity, tunable, and coherent X-ray beam. The energy of the X-rays can be varied to probe specific chemical elements or bonding states.
- Focusing Optics: The X-ray beam is focused onto the sample using a Fresnel zone plate, which is a circular diffraction grating that acts as a lens for X-rays. The zone plate focuses the X-rays to a spot size of typically 20-50 nm, enabling high-resolution imaging.
- Sample Stage: The sample is mounted on a high-precision stage that allows for nanometer-scale positioning and scanning. The stage is often equipped with a cryostat or an environmental cell to study samples under different conditions, such as low temperatures or controlled atmospheres.
- Detector: The transmitted X-rays are detected using a high-sensitivity detector, such as a photomultiplier tube or a charged-coupled device (CCD). The detector records the intensity of the transmitted X-rays at each scan position, forming a 2D image of the sample.
Imaging Modes in STXM
STXM offers several imaging modes that provide complementary information about the sample:
Absorption Contrast Imaging
In absorption contrast imaging, the contrast in the STXM image arises from differences in the X-ray absorption of the sample. Chemical elements with higher atomic numbers or higher concentrations absorb X-rays more strongly, appearing darker in the image. This mode is useful for mapping the distribution of chemical elements or phases in the sample.
Spectromicroscopy
Spectromicroscopy involves acquiring a series of STXM images at different X-ray energies, typically across an absorption edge of a specific chemical element. By analyzing the changes in the absorption spectra at each pixel, it is possible to obtain detailed information about the chemical state, oxidation state, and local bonding environment of the element of interest. This mode is particularly useful for studying complex, heterogeneous materials with nanoscale chemical variations.
Differential Phase Contrast Imaging
Differential phase contrast imaging exploits the phase shift of the X-rays as they pass through the sample. This mode is sensitive to variations in the refractive index of the sample, providing contrast based on the material's electron density. Differential phase contrast imaging is useful for studying weakly absorbing samples or materials with similar absorption properties.
Applications of STXM
STXM has found widespread applications in various fields, including materials science, nanotechnology, chemistry, and biology:
- Nanomaterials Characterization: STXM is extensively used to study the chemical composition, phase distribution, and electronic structure of nanomaterials, such as nanoparticles, nanowires, and thin films. It provides valuable insights into the structure-property relationships of these materials at the nanoscale.
- Polymer Science: STXM is a powerful tool for investigating the morphology, phase separation, and chemical composition of polymeric materials. It can reveal the nanoscale distribution of different polymer components, additives, or fillers, helping to understand the structure and performance of polymer blends and composites.
- Environmental Science: STXM is employed to study the fate and transport of nanomaterials in the environment, such as the interaction of nanoparticles with natural organic matter or their uptake by organisms. It provides insights into the biogeochemical processes and potential environmental impacts of nanomaterials.
- Biological Imaging: STXM is increasingly used in biological and biomedical research to image cells, tissues, and biomolecules at the nanoscale. It can map the distribution of specific chemical elements or functional groups, such as proteins, lipids, or DNA, within biological samples, providing valuable information about their structure and function.
Advantages and Limitations of STXM
STXM offers several advantages over other imaging techniques:
- High spatial resolution, typically in the range of 20-50 nm
- Chemical sensitivity and elemental specificity
- Non-destructive imaging of sensitive or beam-sensitive samples
- Ability to study samples under various environmental conditions
However, STXM also has some limitations:
- Requires access to a synchrotron radiation facility
- Limited sample thickness, typically a few hundred nanometers
- Relatively long acquisition times for spectromicroscopy measurements
Future Developments in STXM
Ongoing developments in STXM aim to enhance its capabilities and expand its applications. Some of the key areas of research include:
- Improving the spatial resolution by developing advanced focusing optics, such as multilayer Laue lenses or ptychographic imaging methods
- Increasing the temporal resolution for dynamic studies of nanoscale processes, such as chemical reactions or phase transformations
- Combining STXM with other complementary techniques, such as X-ray fluorescence or X-ray diffraction, to obtain a more comprehensive understanding of the sample
- Developing new sample environments and in situ cells for studying materials under realistic operating conditions, such as in batteries, fuel cells, or catalytic reactions
As STXM continues to evolve, it is expected to play an increasingly important role in unraveling the complexities of the nanoscale world and advancing the development of new materials and technologies.
Further Reading
Journal of Electron Spectroscopy and Related Phenomena, Scanning transmission X-ray microscopy at the Advanced Light Source
Review of Scientific Instruments, Cryo scanning transmission x-ray microscope optimized for spectrotomography
Journal of Electron Spectroscopy and Related Phenomena, Energy material analysis via in-situ/operando scanning transmission x-ray microscopy: A review