Nucleic Acids: The Building Blocks of Life
What are Nucleic Acids?
Nucleic acids are complex biological molecules that store and transmit genetic information in all living organisms. They are essential for the survival and reproduction of life on Earth. The two main types of nucleic acids are deoxyribonucleic acid (DNA) and ribonucleic acid (RNA).
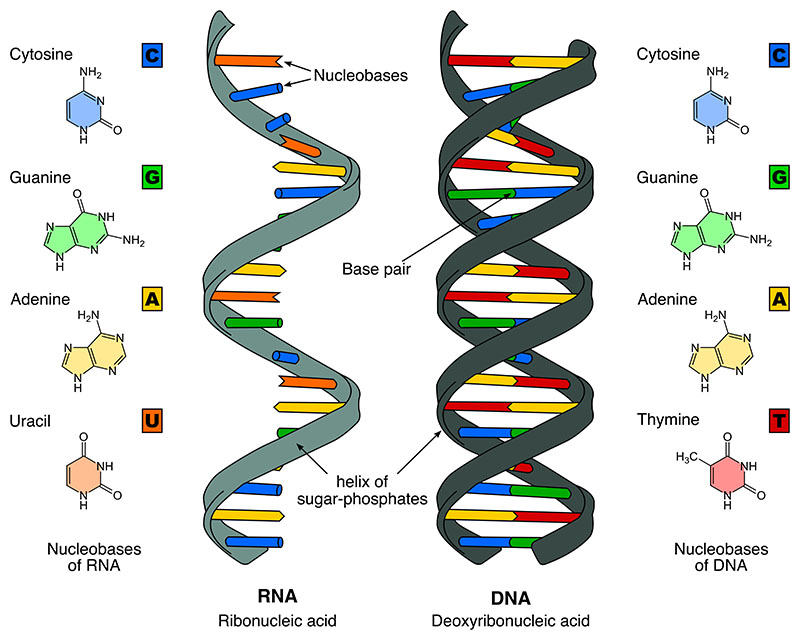
Structure and Composition of Nucleic Acids
Nucleic acids are polymers composed of repeating units called nucleotides. Each nucleotide consists of three components:
- Sugar: In DNA, the sugar is deoxyribose, while in RNA, it is ribose. The sugar forms the backbone of the nucleic acid strand.
- Phosphate Group: The phosphate group is attached to the sugar and helps link nucleotides together to form the backbone of the nucleic acid.
- Nitrogenous Base: There are five main nitrogenous bases: adenine (A), guanine (G), cytosine (C), thymine (T), and uracil (U). In DNA, the bases are A, G, C, and T, while in RNA, uracil replaces thymine.
The nucleotides are connected through phosphodiester bonds, forming a long chain. In DNA, two complementary strands of nucleotides pair up through hydrogen bonds between the bases (A with T and G with C) to form the iconic double helix structure. RNA is usually single-stranded but can form complex secondary structures through base pairing within the same strand.
Functions of Nucleic Acids
Nucleic acids play crucial roles in the storage, transmission, and expression of genetic information:
DNA as the Genetic Blueprint
DNA serves as the repository of genetic information in cells. It encodes the instructions for the synthesis of a proteins and other essential molecules. The specific sequence of nucleotides in DNA determines the genetic traits of an organism and is passed down from parent to offspring during reproduction.
RNA in Gene Expression and Regulation
RNA acts as a messenger and regulator in the process of gene expression. Messenger RNA (mRNA) carries the genetic information from DNA to the ribosomes, where proteins are synthesized. Transfer RNA (tRNA) and ribosomal RNA (rRNA) are involved in the translation of mRNA into proteins.
Various non-coding RNAs also play important roles in regulating gene expression and cellular processes:
- MicroRNAs (miRNAs): Short, non-coding RNAs that regulate gene expression by binding to complementary sequences on target mRNAs, leading to their degradation or translational repression.
- Long non-coding RNAs (lncRNAs): RNA molecules longer than 200 nucleotides that do not encode proteins but have regulatory functions, such as chromatin remodeling and transcriptional regulation.
- Small interfering RNAs (siRNAs): Double-stranded RNAs that trigger the degradation of complementary mRNA through a process called RNA interference (RNAi), effectively silencing gene expression.
- Piwi-interacting RNAs (piRNAs): Small non-coding RNAs that interact with Piwi proteins to silence transposable elements and maintain genome stability, particularly in germline cells.
Replication and Inheritance of Nucleic Acids
The ability of nucleic acids to replicate and pass genetic information from one generation to the next is fundamental to the continuity of life.
DNA Replication
During cell division, DNA undergoes replication to produce two identical copies of the genetic material. The double helix unwinds, and each strand serves as a template for the synthesis of a new complementary strand. This semiconservative replication ensures that each daughter cell receives an exact copy of the genetic information.
RNA Synthesis (Transcription)
The genetic information stored in DNA is transcribed into RNA through the process of transcription. In this process, the enzyme RNA polymerase uses one strand of the DNA as a template to synthesize a complementary RNA strand. The resulting RNA molecule, typically mRNA, carries the genetic message from the nucleus to the cytoplasm for protein synthesis.
Nucleic Acids in Biotechnology and Medicine
The understanding of nucleic acids has revolutionized the fields of biotechnology and medicine, leading to numerous applications:
Genetic Engineering
Nucleic acids are the foundation of genetic engineering techniques, such as recombinant DNA technology and gene editing. By manipulating the genetic material, scientists can create genetically modified organisms (GMOs) with desired traits, produce therapeutic proteins, and develop new crop varieties.
Molecular Diagnostics
Nucleic acid-based techniques, such as polymerase chain reaction (PCR) and DNA sequencing, are widely used in molecular diagnostics. These methods allow for the detection and identification of pathogens, genetic disorders, and cancer biomarkers, enabling early diagnosis and targeted treatment.
Gene Therapy
Gene therapy involves the introduction of functional genes into cells to replace or correct defective genes. Nucleic acids, such as viral vectors or synthetic nucleic acid molecules, are used to deliver the therapeutic genes into target cells. Gene therapy holds promise for treating genetic disorders, cancer, and other diseases.
Nucleic Acid Vaccines
Nucleic acid vaccines, such as mRNA and DNA vaccines, have emerged as a promising approach to immunization. These vaccines deliver genetic instructions to host cells, directing them to produce specific antigens that elicit an immune response. The rapid development and success of mRNA vaccines against COVID-19 have highlighted the potential of this technology.
Challenges and Future Perspectives
Despite the remarkable progress in understanding and manipulating nucleic acids, challenges remain. One of the main challenges is the delivery of nucleic acids into cells efficiently and safely. Researchers are developing novel delivery systems, such as nanoparticles and viral vectors, to overcome this hurdle.
The future of nucleic acid research lies in unraveling the complexities of gene regulation, exploring the roles of non-coding RNAs, and developing personalized therapies based on individual genetic profiles. The integration of genomics, transcriptomics, and proteomiproteomicscs data will provide a comprehensive understanding of biological systems and enable the development of precise diagnostic and therapeutic strategies.
Further Reading
International Journal of Molecular Sciences, Advances in Nucleic Acid Research: Exploring the Potential of Oligonucleotides for Therapeutic Applications and Biological Studies
Nature Nanotechnology, The current landscape of nucleic acid therapeutics
Advanced Drug Delivery Reviews, Nucleic acid delivery for therapeutic applications